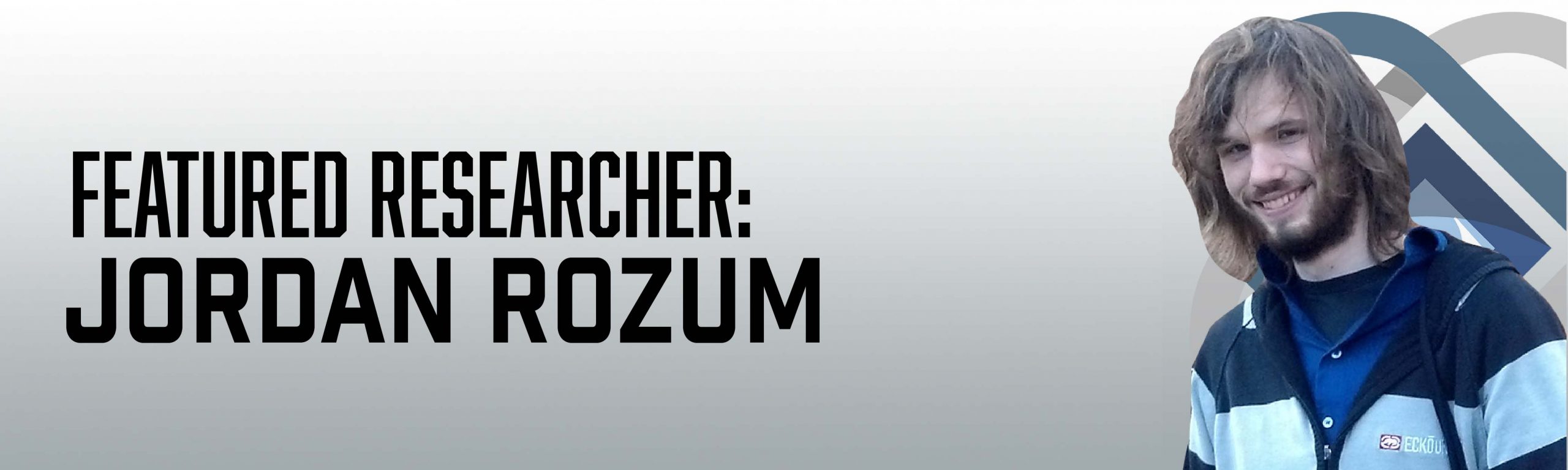
Featured Researcher: Jordan Rozum
Posted on September 15, 2021Cracking the code of whole genome-scale models could lead to cutting-edge medical treatments for diseases like cancer and gain new insights into biology and health. However, researchers face computational problems probing the whole genome models because of their incredible complexity. Jordan is hoping to find ways to reduce this vast sea of possibilities to help scientists better understand the genome. All of this requires a lot of computing power, and that is where ICDS comes in.
How did you get into this research field?
I used to study differential geometry and general relativity, and I got my master’s degree in mathematics in that field. I made the switch to biological physics while I was a student at the University of Illinois at Urbana Champaign. I was exploring ways to analyze biological data that involve thinking of datapoints embedded in a curved space. While investigating these possibilities, I came across the field of network biophysics, and was hooked. I eventually transferred here, to Penn State, where I have been studying networks ever since.
What do you hope to accomplish with your research?
The human genome has around 20,000 protein-coding genes. Their patterns of activity and inactivity govern the form and function of the cells in your body, and our goal is to better understand these patterns. How do they arise? How can we manipulate them to help cure or treat complex diseases like cancer? These are big questions, and they can only be tackled when many researchers approach them from many different angles. Our approach is to study gene regulation as a dynamics problem constrained by a network of known interactions between genes. Specifically, we are interested in identifying the long-term behaviors (i.e., the stable phenotypes) of these systems and in figuring out how to select one phenotype over another using, for example, pharmaceutical drugs.
How does supercomputing enable your research?
We often study small pieces of the gene regulatory network that governs all cells, focusing our attention on regulatory circuits that play key roles in specific biological processes, like cell division, programmed cell death, or cancer metastasis. Despite this narrow focus, we often need to consider several dozen genes at once in a given model. Larger models can have hundreds or even thousands of variables. Even if we assume that a gene can only be in one of two states – active or inactive – the number of possible configurations is literally astronomical. With only a few hundred variables, there are already more configurations than there are atoms in the observable universe. The number of configurations grows exponentially with the number of variables. Recently, we have been interested in whole-genome-scale models, which contain tens of thousands of variables. Identifying the stable long-term behaviors within this vast sea of possibilities requires careful reasoning to reduce the search space and a lot of computing power to sift through what remains.
What is your academic background?
I got my bachelor’s degree in math and physics and my master’s degree in math from Utah State University. I studied astrophysics and the mathematics of general relativity. A brief detour into applications of the math of curved space-time to bioinformatics eventually became a complete shift in my focus. I am now nearing completion of my PhD in physics here at Penn State where I study the mathematics of network models of biological processes.
What important advances do you think we’ll see in your field in five years? Ten? Twenty?
The process of developing models of cancer and other complex diseases on a cellular level is becoming increasingly streamlined. At the same time, people across the world are already working on ways to quickly build personalized, multi-scale models of complex diseases. Together with the increasing availability of genetic testing, this has the potential to radically change how potential treatments for various cancers are explored, not just at the R&D level at big pharmaceutical companies, but in the doctor’s office. I expect that over the next several years and decades modeling and genetic testing will play an increasingly important role in developing personalized treatment plans for individual patients with cancer or other diseases.
Share
Related Posts
- Featured Researcher: Nick Tusay
- Multi-institutional team to use AI to evaluate social, behavioral science claims
- NSF invests in cyberinfrastructure institute to harness cosmic data
- Center for Immersive Experiences set to debut, serving researchers and students
- Distant Suns, Distant Worlds
- CyberScience Seminar: Researcher to discuss how AI can help people avoid adverse drug interactions
- AI could offer warnings about serious side effects of drug-drug interactions
- Taking RTKI drugs during radiotherapy may not aid survival, worsens side effects
- Cost-effective cloud research computing options now available for researchers
- Costs of natural disasters are increasing at the high end
- Model helps choose wind farm locations, predicts output
- Virus may jump species through ‘rock-and-roll’ motion with receptors
- Researchers seek to revolutionize catalyst design with machine learning
- Resilient Resumes team places third in Nittany AI Challenge
- ‘AI in Action’: Machine learning may help scientists explore deep sleep
- Clickbait Secrets Exposed! Humans and AI team up to improve clickbait detection
- Focusing computational power for more accurate, efficient weather forecasts
- How many Earth-like planets are around sun-like stars?
- Professor receives NSF grant to model cell disorder in heart
- SMH! Brains trained on e-devices may struggle to understand scientific info
- Whole genome sequencing may help officials get a handle on disease outbreaks
- New tool could reduce security analysts’ workloads by automating data triage
- Careful analysis of volcano’s plumbing system may give tips on pending eruptions
- Reducing farm greenhouse gas emissions may plant the seed for a cooler planet
- Using artificial intelligence to detect discrimination
- Four ways scholars say we can cut the chances of nasty satellite data surprises
- Game theory shows why stigmatization may not make sense in modern society
- Older adults can serve communities as engines of everyday innovation
- Pig-Pen effect: Mixing skin oil and ozone can produce a personal pollution cloud
- Researchers find genes that could help create more resilient chickens
- Despite dire predictions, levels of social support remain steady in the U.S.
- For many, friends and family, not doctors, serve as a gateway to opioid misuse
- New algorithm may help people store more pictures, share videos faster
- Head named for Ken and Mary Alice Lindquist Department of Nuclear Engineering
- Scientific evidence boosts action for activists, decreases action for scientists
- People explore options, then selectively represent good options to make difficult decisions
- Map reveals that lynching extended far beyond the deep South
- Gravitational forces in protoplanetary disks push super-Earths close to stars
- Supercomputer cluster donation helps turn high school class into climate science research lab
- Believing machines can out-do people may fuel acceptance of self-driving cars
- People more likely to trust machines than humans with their private info
- IBM donates system to Penn State to advance AI research
- ICS Seed Grants to power projects that use AI, machine learning for common good
- Penn State Berks team advances to MVP Phase of Nittany AI Challenge
- Creepy computers or people partners? Working to make AI that enhances humanity
- Sky is clearing for using AI to probe weather variability
- ‘AI will see you now’: Panel to discuss the AI revolution in health and medicine
- Privacy law scholars must address potential for nasty satellite data surprises
- Researchers take aim at hackers trying to attack high-value AI models
- Girls, economically disadvantaged less likely to get parental urging to study computers
- Seed grants awarded to projects using Twitter data
- Researchers find features that shape mechanical force during protein synthesis
- A peek at living room decor suggests how decorations vary around the world
- Interactive websites may cause antismoking messages to backfire
- Changing how government assesses risk may ease fallout from extreme financial events
- Differences in genes’ geographic origin influence mitochondrial function
- ICS Affiliate named AGU fellow
- ICS co-sponsors Health, Environment Seed Grant Program